Just a year ago, Peter Higgs and Francois Engler received the Nobel Prize for their work on subatomic particles. This may seem ridiculous, but scientists made their discoveries half a century ago, but to this day they have not been given any significant significance.
In 1964, two more talented physicists also appeared with their pioneering theory. At first, she also attracted almost no attention. This is strange, since she described the structure of hadrons, without which no strong interatomic interaction is possible. It was a theory of quarks.
What it is?
By the way, what is a quark? This is one of the most important components of a hadron. Important! This particle has a “half” spin, actually being a fermion. Depending on the color (see below), the quark charge can be equal to one third or two thirds of the proton charge. As for the flowers, there are six of them (generations of quarks). They are needed so that the Pauli principle is not violated.
Basic information
In the composition of hadrons, these particles are at a distance not exceeding the value of confinement. The explanation is simple: they exchange vectors of the gauge field, that is, gluons. Why is the quark so important? Gluon plasma (saturated with quarks) is the state of matter in which the entire universe was located immediately after the big bang. Accordingly, the existence of quarks and gluons is a direct confirmation of what it really was.
They also have their own color, and therefore during the movement they create their own virtual copies. Accordingly, with increasing distance between quarks, the force of interaction between them increases significantly. As you might guess, with a minimum distance, the interaction practically disappears (asymptotic freedom).
Thus, any strong interaction in hadrons is explained by the transition of gluons between quarks. If we talk about the interactions between hadrons, then they are explained by the transfer of pi-meson resonance. Simply put, indirectly everything again comes down to gluon exchange.
How many quarks are there in nucleons?
Each neutron consists of a pair of d-quarks, as well as a single u-quark. Each proton, on the contrary, is from a single d-quark and a pair of u-quarks. By the way, letters are placed depending on quantum numbers.
Let’s explain. For example, beta decay is explained just by the same transformation of one of the same type of quarks in the nucleon into another. To better understand, in the form of a formula this process can be written like this: d = u + w (this is neutron decay). Accordingly, the proton is written in a slightly different formula: u = d + w.
Incidentally, it is the latter process that explains the constant flux of neutrinos and positrons from large stellar clusters. So, on the scale of the Universe, there are few such important particles as a quark: gluon plasma, as we have already said, confirms the fact of a big explosion, and studies of these particles allow scientists to better know the very essence of the world in which we live.
What is less than a quark?
By the way, what are quarks made of? Their constituent particles are preons. These particles are very small and poorly studied, so even today not much is known about them. Here's what's less than a quark.
Where did they come from?
To date, two hypotheses for the formation of preons are most common: string theory and the Bilson-Thompson theory. In the first case, the occurrence of these particles is explained by the oscillation of the strings. The second hypothesis suggests that their appearance is caused by an excited state of space and time.
Interestingly, in the second case, the phenomenon can be completely described using the parallel transport matrix along the curves of the spin network. The properties of this matrix itself determine those for preon. This is what quarks are made of.
Summing up some results, we can say that quarks are a kind of "quanta" in the composition of hadrons. Are you impressed? And now we’ll talk about how the quark was opened. This is a very fascinating story, which, among other things, more fully reveals some of the nuances described above.
Strange particles
Immediately after the end of World War II, scientists began to actively explore the world of subatomic particles, which until then looked primitive simply (according to those ideas). Protons, neutrons (nucleons) and electrons formed an atom. In 1947, peonies were discovered (and predicted their existence back in 1935), which were responsible for the mutual attraction of nucleons in the nucleus of atoms. At the time, more than one scientific exhibition was dedicated to this event. The quarks had not yet been opened, but the moment of the attack on their “trace” was getting closer.
The neutrinos were not yet open by then. But their obvious importance for explaining the beta decays of atoms was so great that scientists had little doubt about their existence. In addition, some antiparticles have already been detected or predicted. It remained unclear only the situation with muons, which were formed during the decay of pions and subsequently transferred to the state of a neutrino, electron, or positron. Physicists did not understand why this intermediate station was needed at all .
Alas, such a simple and unpretentious model very briefly survived the moment of discovery of peonies. In 1947, two English physicists, George Rochester and Clifford Butler, published a curious article in the scientific journal Nature. The material for her was their study of cosmic rays through a Wilson camera, during which they received curious information. In one of the photographs taken during the observation process, a pair of tracks with a common beginning was clearly visible. Since the discrepancy was reminiscent of the Latin V, it immediately became clear that the charge of these particles was definitely different.
Scientists immediately suggested that these tracks indicate the fact of the decay of some unknown particle, which left no other traces. Calculations showed that its mass is about 500 MeV, which is much more than this value for an electron. Of course, the researchers called their discovery a V-particle. However, it was not a quark yet. This particle was still waiting in the wings.
This is just the beginning
With this discovery, it all started. In 1949, under the same conditions, a trace of a particle was discovered, which gave rise immediately to three pions. It soon became clear that it, like the V-particle, are completely different representatives of the four-particle family. Subsequently, they were called K-mesons (kaons).
A pair of charged kaons have a mass of 494 MeV, and in the case of a neutral charge, 498 MeV. By the way, in 1947, scientists were lucky to capture just the very rare case of the decay of the positive kaon, but at that time they simply could not correctly interpret the picture. However, to be completely fair, in fact, the first observation of the kaon was made back in 1943, but information about this was almost lost against the background of numerous post-war scientific publications.
New oddities
And then more discoveries awaited scientists. In 1950 and 1951, researchers from the University of Manchester and Melbourne managed to find particles much heavier than protons and neutrons. She again had no charge, but decayed into a proton and a pion. The latter, as can be understood, had a negative charge. The new particle was designated by the letter Λ (lambda).
The more time passed, the more questions arose from scientists. The problem was that new particles appeared exclusively during strong atomic interactions, quickly decaying into known protons and neutrons. In addition, they always appeared in pairs, there were never single manifestations. That is why a group of physicists from the USA and Japan suggested using a new quantum number in their description - strangeness. According to their definition, the oddity of all other known particles was zero.
Further research
A breakthrough in research occurred only after the emergence of a new systematization of hadrons. The most prominent figure in this was the Israeli Juval Neeman, who changed the career of an outstanding military man to the equally brilliant path of a scientist.
He drew attention to the fact that mesons and baryons open by that time were decaying, forming a cluster of related particles, multiplets. The members of each such association have exactly the same strangeness, but opposite electrical charges. Since really strong nuclear interactions do not depend on electric charges at all, in everything else, particles from a multiplet look like perfect twins.
Scientists have suggested that some kind of natural symmetry is responsible for the occurrence of such formations, and soon they managed to find it. It turned out to be a simple generalization of the spin group SU (2), which scientists all over the world used to describe quantum numbers. But only by that time 23 hadrons were already known, and their spins were equal to 0, ½ or a whole unit, and therefore it was not possible to use such a classification.
As a result, it was necessary to use two quantum numbers for classification at once, due to which the classification expanded significantly. So the SU group (3) appeared, which at the beginning of the century was created by the French mathematician Eli Cartan. To determine the systematic position of each particle in it, the scientists developed a research program. Quark subsequently easily entered the systematic series, which confirmed the absolute correctness of the specialists.
New quantum numbers
So scientists came up with the idea of ​​using abstract
quantum numbers, which became hypercharge and isotopic spin. However, with the same success we can take strangeness and electric charge. This scheme was conditionally called the
Eightfold Path. This captures the analogy with Buddhism, where eight levels are also required before reaching nirvana. However, all this is lyrics.
Neeman and his colleague, Gell-Mann, published their works in 1961, and the number of mesons known then did not exceed seven. But in their work, the researchers were not afraid to mention the high probability of the existence of the eighth meson. In the same 1961, their theory was brilliantly confirmed. The found particle was called eta-meson (Greek letter η).
Further findings and brilliance experiments confirmed the absolute correctness of the SU classification (3). This circumstance was a powerful incentive for researchers who found themselves on the right track. Even Gell-Mann himself no longer doubted that quarks exist in nature. Reviews of his theory were not very positive, but the scientist was confident that he was right.
Here are the quarks!
Soon the article “Schematic model of baryons and mesons” was published. In it, scientists were able to further develop the idea of ​​systematization, which turned out to be so useful. They found that SU (3) fully admits the existence of whole triplets of fermions, the electric charge of which ranges from 2/3 to 1/3 and –1/3, and in a triplet one particle always differs in nonzero strangeness. Gell-Mann, already well-known to us, called them "elementary particles of a quark."
According to the charges, he designated them as u, d and s (from the English words up, down and strange). In accordance with the new scheme, each baryon is formed immediately by three quarks. Mesons are much simpler. They include one quark (this rule is unshakable) and an antiquark. Only then did the scientific community become aware of the existence of these particles, which our article is dedicated to.
A little more background
This article, which in many respects predetermined the development of physics for years to come, has a rather curious background. Gell-Mann thought about the existence of such triplets long before its publication, but did not discuss his assumptions with anyone. The fact is that his assumptions about the existence of particles with a fractional charge looked like nonsense. However, after a conversation with the prominent theoretical physicist Robert Serber, he found out that his colleague had made exactly the same conclusions.
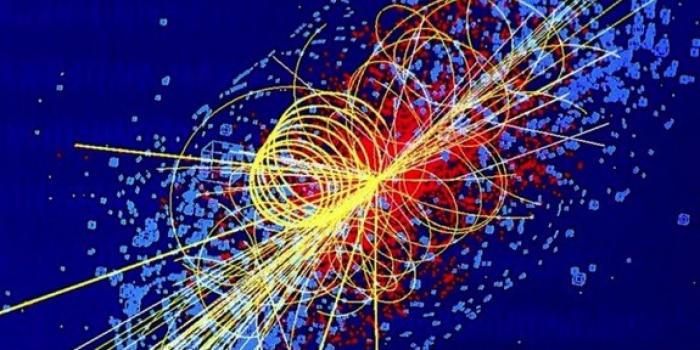
In addition, the scientist made the only correct conclusion: the existence of such particles is possible only if they are not free fermions, but are part of hadrons. Indeed, in this case, their charges make up a single whole! At first, Gell-Mann called them quarks and even mentioned them in the MTI, but the reaction of students and teachers was very restrained. And so the scientist thought for a very long time about whether he should submit his research to the public.
The very word “quark” (a sound reminiscent of the cry of ducks) was taken from the work of James Joyce. Oddly enough, but the American scientist sent his article to the prestigious European scientific journal Physics Letters, as he seriously feared that the editors of a similar level American publication Physical Review Letters would not accept it for publication. By the way, if you want to look at least at least a copy of that article, you have a direct road to the same Berlin museum. There are no quarks in his exposition, but there is a complete history of their discovery (more precisely, documentary evidence).
The beginning of the quark revolution
In fairness, it is worth noting that almost at the same time, a scientist from CERN, George Zweig, reached a similar thought. At first, Gell-Mann himself was his mentor, and then Richard Feynman. Zweig also determined the reality of the existence of fermions that possessed fractional charges, only called them aces. Moreover, the talented physicist also considered baryons as a triple of quarks, and mesons as a combination of a quark and an antiquark.
Simply put, the student completely repeated the conclusions of his teacher, and completely separate from him. His work appeared even a couple of weeks before Mann’s publication, but only as a “homework” of the institute. However, it was the presence of two independent works, the conclusions on which were almost identical, immediately convinced some scientists that the proposed theory was correct.
From rejection to trust
But many researchers did not immediately accept this theory. Yes, journalists and theorists quickly fell in love with it for clarity and simplicity, but serious physicists accepted it only after a whole 12 years. Do not blame them for excessive conservatism. The fact is that initially the theory of quarks sharply contradicted the Pauli principle, which we mentioned at the very beginning of the article. If we assume that the proton contains a pair of u-quarks and a single d-quark, then the first should be strictly in the same quantum state. According to Pauli, this is impossible.
It was then that an additional quantum number appeared, expressed in the form of color (which we also mentioned above). In addition, it was completely incomprehensible how generally elementary particles of quarks interact with each other, why their free varieties are not found. All these secrets were greatly helped by the Theory of Gauge Fields, which was "brought to mind" only in the mid-70s. At about the same time, the quark theory of hadrons was organically incorporated into it.
But the development of the theory was most hindered most by the complete absence of at least some experimental experiments that would confirm both the very existence and the interaction of quarks with each other and with other particles. And they gradually began to appear only in the late 60s, when the rapid development of technology made it possible to conduct an experiment with the "transmission" of protons by electron fluxes. It was these experiments that made it possible to prove that inside the protons some particles that were originally called partons really “hide”. Subsequently, everyone was convinced that this was nothing but a true quark, but this only happened at the end of 1972.
Experimental confirmation
Of course, for the final conviction of the scientific community, much more experimental data was required. In 1964, James Bjorken and Sheldon Glashow (future Nobel Prize winner, by the way) made the assumption that there might be a fourth kind of quark, which they called charmed.
Thanks to this hypothesis, scientists in 1970 were able to explain many of the oddities that were observed during the decay of neutrally charged kaons. Four years later, two independent groups of American physicists were able to fix the decay of the meson, which included just one “enchanted” quark, as well as its antiquark. It is not surprising that this event was immediately dubbed the November Revolution. For the first time, the theory of quarks received more or less "visual" confirmation.
The importance of the discovery is evidenced even by the fact that the project managers, Samuel Thing and Barton Richter, accepted their Nobel Prize two years later: this event is reflected in many articles. You can familiarize yourself with some of them in the original if you visit the New York Museum of Natural Science. Quarks, as we have already said, are an extremely important discovery of modernity, and therefore a lot of attention is paid to them in the scientific community.
Last argument
Only in 1976, researchers still found one particle with nonzero charm, a neutral D-meson. This is a rather complicated combination of one enchanted quark and u-antique. Here, even inveterate opponents of the existence of quarks were forced to admit the correctness of the theory, first set forth more than two decades ago. One of the most famous theoretical physicists, John Ellis, called the charm "the lever that turned the world."
Soon, a list of new discoveries also included a pair of especially massive quarks, top and bottom, which were easily able to be correlated with the SU systematization (3) already adopted at that time. In recent years, scientists have said that there are so-called tetraquarks, which some scientists have already dubbed "hadron molecules."
Some conclusions and conclusions
It must be understood that the discovery and scientific justification of the existence of quarks can indeed be safely considered a scientific revolution. It can be considered the beginning of 1947 (in principle, 1943), and its end falls on the discovery of the first "enchanted" meson. It turns out that the duration of the last opening of this level to date is no less than 29 years (or even 32 years)! And all this time was spent not only in order to find a quark! Gluon plasma as the primary object in the Universe soon attracted much more attention of scientists.
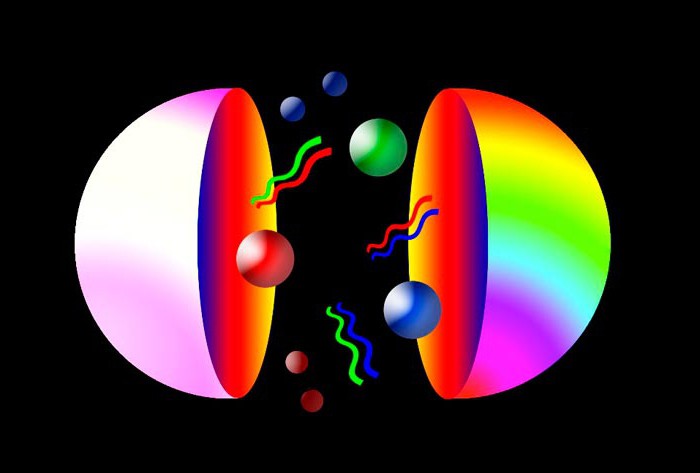
However, the more difficult the field of study becomes, the more time is required to make truly important discoveries. As for the particles we are discussing, the importance of such a discovery cannot be underestimated by anyone. By studying the structure of quarks, a person will be able to penetrate deeper into the secrets of the universe. It is possible that only after a complete study of them, we can find out how the big explosion occurred and by what laws our Universe is developing. In any case, it was their discovery that convinced many physicists that the reality surrounding us was much more complicated than past ideas.
So you find out what a quark is. This particle in its time made a lot of noise in the scientific world, and even today, researchers are full of hope to finally reveal all its secrets.