X-rays have a number of unique properties as radiation that go beyond their very short wavelength. One of their important properties for science is element selectivity. Choosing and studying the spectra of individual elements that are located in unique places in complex molecules, we have a localized “atomic sensor”. Investigating these atoms at different times after the structure is excited by light, we can trace the development of electronic and structural changes even in very complex systems, or, in other words, we can follow an electron through a molecule and across interfaces.
History
The inventor of radiography was Wilhelm Conrad Röntgen. Once, when a scientist examined the ability of various materials to stop rays, he placed a small piece of lead in position while a discharge occurred. Thus, X-ray saw the first radiographic image, his own shimmering ghostly skeleton on the screen of barium platinum cyanide. He later said that it was at that moment that he decided to continue his experiments in secret, because he was afraid for his professional reputation if his observations were erroneous. The German scientist was awarded the first Nobel Prize in physics in 1901 for the discovery of X-rays in 1895. According to the National SLAC Accelerator Laboratory, its new technology was quickly used by other scientists and doctors.
Charles Barkla, a British physicist, conducted research between 1906 and 1908, which led to his discovery that x-rays could be characteristic of individual substances. His work also earned him the Nobel Prize in physics, but only in 1917.
The use of X-ray spectroscopy actually began a little earlier, in 1912, starting with the collaboration of the father and son of British physicists, William Henry Bragg (William Henry Bragg) and William Lawrence Bragg (William Lawrence Bragg). They used spectroscopy to study the interaction of x-rays with atoms inside crystals. Their technique, called x-ray crystallography, became the standard in this area by the following year, and they received the Nobel Prize in Physics in 1915.
In action
In recent years, X-ray spectrometry has been used in various new and exciting ways. On the surface of Mars there is an X-ray spectrometer that collects data on the elements that make up the soil. The power of the rays was used to detect lead paint on toys, which reduced the risk of lead poisoning. The partnership between science and art can be seen in the use of radiography, when used in museums to identify elements that can damage collections.
Work principles
When an atom is unstable or is bombarded by high-energy particles, its electrons move between energy levels. When the electrons adapt, the element absorbs and emits high-energy X-ray photons in a manner characteristic of the atoms that make up this particular chemical element. Using X-ray spectroscopy, energy fluctuations can be determined. This allows you to identify particles and see the interaction of atoms in various media.
There are two main methods of X-ray spectroscopy: with wavelength dispersion (WDXS) and with energy dispersion (EDXS). WDXS measures x-rays of the same wavelength that are diffracted on a crystal. EDXS measures the x-rays emitted by electrons stimulated by a high-energy source of charged particles.
Analysis of x-ray spectroscopy in both methods of radiation distribution indicates the atomic structure of the material and, therefore, the elements inside the analyzed object.
X-ray methods
There are several different methods of X-ray and optical spectroscopy of the electronic spectrum that are used in many fields of science and technology, including archeology, astronomy and engineering. These methods can be used independently or together to create a more complete picture of the analyzed material or object.
Wdxs
X-ray photoelectron spectroscopy (WDXS) is a surface-sensitive quantitative spectroscopic method that measures the elemental composition in the range of parts on the surface of the material being studied, and also determines the empirical formula, chemical state and electronic state of the elements that exist in the material. Simply put, WDXS is a useful measurement method because it shows not only which elements are inside the film, but also which elements are formed after processing.
X-ray spectra are obtained by irradiating the material with a beam of X-rays, while measuring the kinetic energy and the number of electrons that leave the upper 0-10 nm of the analyzed material. WDXS requires high vacuum (P ~ 10-8 mbar) or ultra high vacuum (UHV; P <10-9 mbar). Although the WDXS area is currently being developed at atmospheric pressure, in which samples are analyzed under pressure of several tens of millibars.
ESCA (X-ray electron spectroscopy for chemical analysis) is an abbreviation introduced by the research team of Kai Zigban to emphasize the chemical (and not just elementary) information that the method provides. In practice, using typical laboratory X-ray sources, XPS detects all elements with an atomic number (Z) of 3 (lithium) and higher. It cannot easily detect hydrogen (Z = 1) or helium (Z = 2).
Edxs
Energy dispersive X-ray spectroscopy (EDXS) is a chemical microanalysis technique used in combination with scanning electron microscopy (SEM). The EDXS method detects the x-ray radiation emitted by a sample when bombarded by an electron beam to characterize the elemental composition of the analyzed volume. Elements or phases up to 1 μm in size can be analyzed.
When a sample is bombarded by an SEM electron beam, electrons are emitted from the atoms that make up the surface of the sample. The resulting electron voids are filled with electrons from a higher state, and x-ray radiation is emitted to balance the energy difference between the states of the two electrons. X-ray energy is characteristic of the element from which it was emitted.
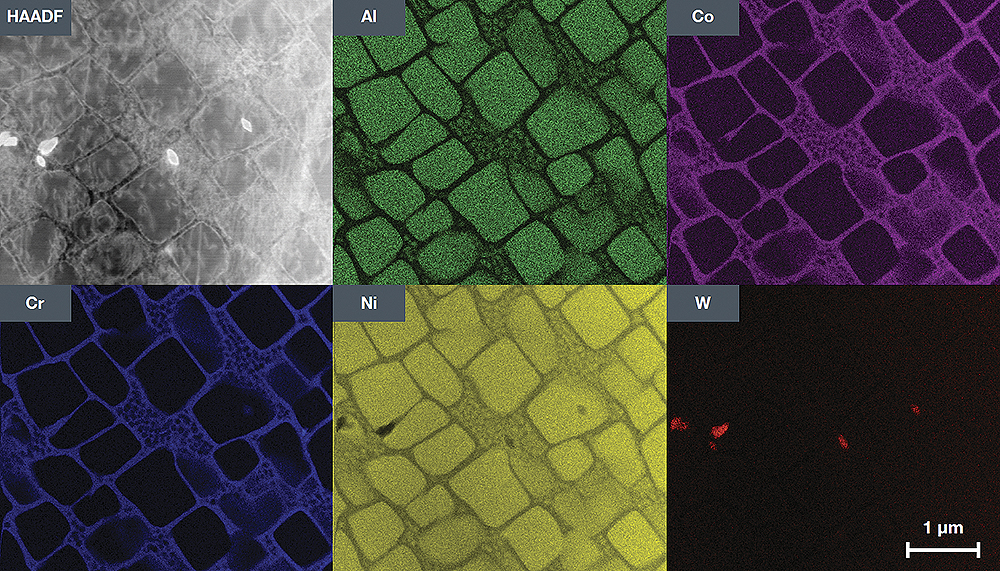
The EDXS X-ray Detector measures the relative amount of emitted rays as a function of their energy. The detector is typically a solid state device with silicon drift lithium. When an incident x-ray hits the detector, it creates a charge pulse, which is proportional to the energy of the x-ray radiation. The charge pulse is converted into a voltage pulse (which remains proportional to the energy of the x-ray radiation) using a charge-sensitive preamplifier. Then the signal is sent to a multi-channel analyzer, where the pulses are sorted by voltage. The energy determined from the voltage measurement for each incident X-ray radiation is sent to a computer to display and further evaluate the data. The X-ray energy spectrum, depending on the count, is estimated to determine the elemental composition of the sample size.
Xrf
X-ray fluorescence spectroscopy (XRF), is used for routine, relatively non-destructive chemical analysis of rocks, minerals, sediments and liquids. Nevertheless, XRF, as a rule, cannot carry out analyzes at small spot sizes (2-5 microns), therefore it is usually used for mass analysis of large fractions of geological materials. The relative lightness and low cost of sample preparation, as well as the stability and ease of use of X-ray spectrometers make this method one of the most widely used for the analysis of the main trace elements in rocks, minerals and sediments.
The physics of XRF X-ray fluorescence spectroscopy depends on fundamental principles that are common to several other instrumental methods, including interactions between electron beams and X-rays with samples, including types of X-ray diffraction, such as SEM-EDS, diffraction (XRD) and wavelength dispersive radiography ( microprobe WDS).
XRF analysis of the main trace elements in geological materials is possible due to the behavior of atoms in their interaction with radiation. When materials are excited by high-energy short-wave radiation (e.g., x-ray radiation), they can become ionized. If the radiation energy is enough to displace a tightly held internal electron, the atom becomes unstable, and the external electron replaces the missing internal. When this happens, energy is released due to the reduced binding energy of the internal electronic orbital compared to the external. Radiation has a lower energy than the primary incident x-ray, and is called fluorescent.
The XRF spectrometer works because if a sample is illuminated by an intense x-ray known as an incident beam, part of the energy is dissipated, but part is also absorbed in the sample, which depends on its chemical composition.
XAS
X-ray absorption spectroscopy (XAS) is the measurement of transitions from the ground electronic states of a metal to excited electronic states (LUMO) and the continuum; the former is known as the near X-ray absorption structure (XANES), and the latter is known as EXAFS, a thin structure with enhanced X-ray absorption, which studies the fine absorption structure at energies above the electron release threshold. These two methods provide additional structural information, the XANES spectra, which report the electronic structure and symmetry of the metal region, and EXAFS, which report the numbers, types, and distances to ligands and neighboring atoms from the absorbing element.
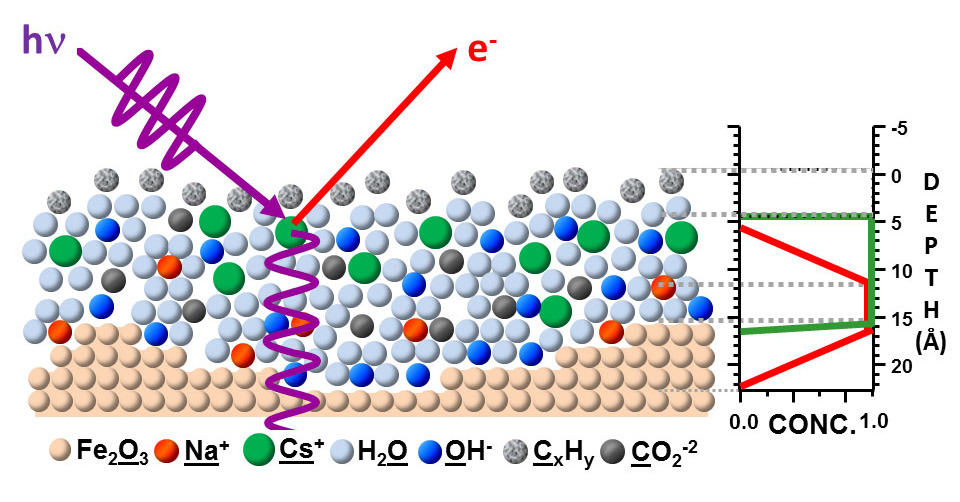
XAS allows us to study the local structure of an element of interest without interference from absorption by a protein matrix, water or air. However, X-ray spectroscopy of metal enzymes was a problem due to the small relative concentration of the element of interest in the sample. In this case, the standard approach was to use X-ray fluorescence to detect absorption spectra instead of using the transmission detection mode. The development of third-generation intensive X-ray sources of synchrotron radiation also made it possible to study diluted samples.
Metal complexes, as models with well-known structures, were necessary for understanding XAS metalloproteins. These complexes provide a basis for assessing the influence of the coordination medium (coordination charge) on the energy of the absorption edge. A study of structurally well characterized model complexes also provides a guideline for understanding EXAFS from metal systems of unknown structure.
A significant advantage of XAS over X-ray crystallography is that local structural information around the element of interest can be obtained even from disordered samples, such as powders and solution. However, ordered samples, such as membranes and single crystals, often increase the information received from XAS. For oriented single crystals or ordered membranes, interatomic vector orientations can be derived from dichroism measurements. These methods are especially useful for determining the structures of multicore metal clusters, such as the Mn4Ca cluster, associated with the oxidation of water in the photosynthetic complex that generates oxygen. Moreover, fairly small changes in the geometry / structure associated with transitions between intermediate states known as S-states in the water oxidation reaction cycle can be easily detected using XAS.
Application methods
X-ray spectroscopy techniques are used in many fields of science, including archeology, anthropology, astronomy, chemistry, geology, engineering, and healthcare. With its help, you can find hidden information about ancient artifacts and remains. For example, Lee Sharp, an associate professor of chemistry at Grinnell College in Iowa, and his colleagues used the XRF method to identify the origins of obsidian arrowheads made by prehistoric people in the North American southwest.
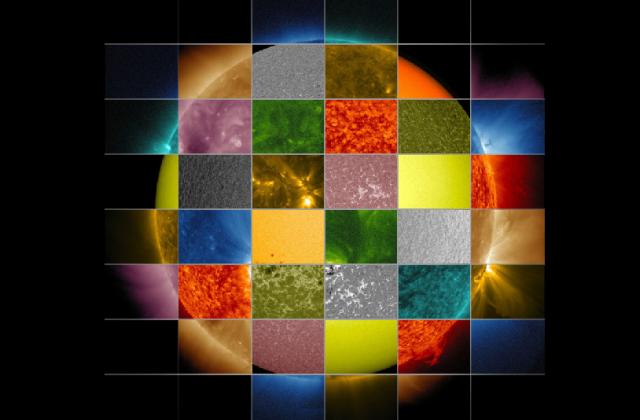
Astrophysicists, thanks to X-ray spectroscopy, will learn more about how objects in space work. So, researchers from the University of Washington in St. Louis plan to observe x-rays from space objects, such as black holes, to learn more about their characteristics. The team, led by Henrik Kravchinsky, an experimental and theoretical astrophysicist, plans to release an X-ray spectrometer called an X-ray polarimeter. Starting in December 2018, the instrument, using a ball filled with helium, was suspended in the atmosphere of the Earth for a long time.
Yuri Gogotsi, a chemist and engineer, uses materials from Drexel University in Pennsylvania to create spray antennas and membranes for desalinating water from materials analyzed by x-ray spectroscopy.
Invisible spray antennas have a thickness of only a few tens of nanometers, but are capable of transmitting and directing radio waves. The XAS technique helps ensure that the composition of the incredibly thin material is correct and helps determine conductivity. “For the antennas to work well, high metal conductivity is required, so we must carefully monitor the material,” Gogotsi said.
Gogotsi and colleagues also use spectroscopy to analyze the surface chemistry of complex membranes that desalinate water by filtering certain ions, such as sodium.
In medicine
X-ray photoelectron spectroscopy is used in several areas of anatomical medical research and in practice, for example, in modern CT scan machines. Collecting X-ray absorption spectra during CT scans (using photon counting or a spectral scanner) can provide more detailed information and determine what is happening inside the body, with lower radiation doses and less or no contrast materials (dyes).